Ununennium- A new element.
- Gandharv Pandey
- Apr 3, 2020
- 10 min read
Ununennium, also known as eka-francium or element 119, is the hypothetical chemical element with symbol Uue and atomic number 119. Ununennium and Uue are the temporary systematic IUPAC name and symbol respectively, which are used until the element is discovered, confirmed, and a permanent name is decided upon. In the periodic table of the elements, it is expected to be an s-block element, an alkali metal, and the first element in the eighth period. It is the lightest element that has not yet been synthesized.
Experiments aimed at the synthesis of ununennium began in June 2018 at RIKEN in Japan; another attempt by the team at the Joint Institute for Nuclear Research at Dubna, Russia began in 2019. Prior to this, two unsuccessful attempts had been made to synthesize ununennium, one by an American team and one by a German team. Theoretical and experimental evidence has shown that the synthesis of ununennium will likely be far more difficult than that of the previous elements, and it may even be the penultimate element that can be synthesized with current technology.
Ununennium's position as the seventh alkali metal suggests that it would have similar properties to its lighter congeners: lithium, sodium, potassium, rubidium, caesium, and francium. However, relativistic effects may cause some of its properties to differ from those expected from a straight application of periodic trends. For example, ununennium is expected to be less reactive than caesium and francium and to be closer in behavior to potassium or rubidium, and while it should show the characteristic +1 oxidation state of the alkali metals, it is also predicted to show the +3 oxidation state, which is unknown in any other alkali metal.
History
Superheavy elements are produced by nuclear fusion. These fusion reactions can be divided into "hot" and "cold" fusion,[a] depending on the excitation energy of the compound nucleus produced. In hot fusion reactions, very light, high-energy projectiles are accelerated toward very heavy targets (actinides), giving rise to compound nuclei at high excitation energy (~40–50 MeV) that may fission, or alternatively evaporate several (3 to 5) neutrons.[9] In cold fusion reactions (which use heavier projectiles, typically from the fourth period, and lighter targets, usually lead and bismuth), the fused nuclei produced have a relatively low excitation energy (~10–20 MeV), which decreases the probability that these products will undergo fission reactions. As the fused nuclei cool to the ground state, they require emission of only one or two neutrons. However, hot fusion reactions tend to produce more neutron-rich products because the actinides have the highest neutron-to-proton ratios of any elements that can presently be made in macroscopic quantities.[10]
Ununennium and unbinilium (elements 119 and 120) are the elements with the lowest atomic numbers that have not yet been synthesized. Attempts to synthesize them would push the limits of current technology, due to the decreasing cross sections of the production reactions and their probably short half-lives,[11] expected to be on the order of microseconds.[1][7] Elements beyond unbiunium (element 121) would likely be too short-lived to be detected with current technology: they would decay within a microsecond, be
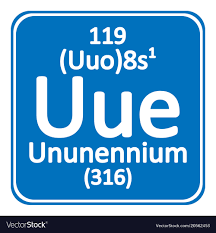
fore reaching the detectors. The possibility of detection of elements 121 through 124 depends greatly on the theoretical model being used, as their half-lives are predicted to be very close to the one-microsecond border.[11] Previously, important help (characterized as "silver bullets") in the synthesis of superheavy elements came from the deformed nuclear shells around hassium-270 which increased the stability of surrounding nuclei, and the existence of the quasi-stable neutron-rich isotope calcium-48 which could be used as a projectile to produce more neutron-rich isotopes of superheavy elements.[12] The more neutron-rich a superheavy nuclide is, the closer it is expected to be to the sought-after island of stability.[b] Even so, the synthesized isotopes still have fewer neutrons than those expected to be in the island of stability.[15] Furthermore, using calcium-48 to synthesize ununennium would require a target of einsteinium-253 or -254, which are very difficult to produce in sufficiently large quantities (only micrograms are presently available; in comparison, milligrams of berkelium and californium are available). More practical production of further super heavy elements would require projectiles heavier than 48Ca.[12]
Synthesis attempts
Past
The synthesis of ununennium was first attempted in 1985 by bombarding a target of einsteinium-254 with calcium-48 ions at the superHILAC accelerator at Berkeley, California:
254 99Es + 48 20Ca → 302 119Uue * → no atoms
No atoms were identified, leading to a limiting cross section of 300 nb. Later calculations suggest that the cross section of the 3n reaction (which would result in 299Uue and three neutrons as products) would actually be six hundred thousand times lower than this upper bound, at 0.5 pb.
As ununennium is the lightest undiscovered element, it has been the target of synthesis experiments by German, Russian, and Japanese teams in recent years. The Russian team at the Joint Institute for Nuclear Research in Dubna, Russia planned to conduct an experiment before 2012, and no results were released, strongly implying that either the experiment was not done or no ununennium atoms were identified. From April to September 2012, an attempt to synthesize the isotopes 295Uue and 296Uue was made by bombarding a target of berkelium-249 with titanium-50 at the GSI Helmholtz Centre for Heavy Ion Research in Darmstadt, Germany.[18][19] Based on the theoretically predicted cross section, it was expected that an ununennium atom would be synthesized within five months of the beginning of the experiment.[11]
249 97Bk + 50 22Ti → 299 119Uue * → 296 119Uue + 3 1 0 n
249 97Bk + 50 22Ti → 299 119Uue * → 295 119Uue + 4 1 0 n
The experiment was originally planned to continue to November 2012, but was stopped early to make use of the 249Bk target to confirm the synthesis of tennessine (thus changing the projectiles to 48Ca). This reaction between 249Bk and 50Ti was predicted to be the most favorable practical reaction for formation of ununennium, as it is rather asymmetrical, though also somewhat cold. (The reaction between 254Es and 48Ca would be superior, but preparing milligram quantities of 254Es for a target is difficult). Nevertheless, the necessary change from the "silver bullet" 48Ca to 50Ti divides the expected yield of ununennium by about twenty, as the yield is strongly dependent on the asymmetry of the fusion reaction.
Due to the predicted short half-lives, the GSI team used new "fast" electronics capable of registering decay events within microseconds. No ununennium atoms were identified, implying a limiting cross section of 70 fb. The predicted actual cross section is around 40 fb, which is at the limits of current technology. (The record lowest cross section of an experimentally successful reaction is 30 fb for the reaction between 209Bi and 70Zn producing nihonium.)
Present
The team at RIKEN in Wakō, Japan began bombarding curium-248 targets with a vanadium-51 beam in June 2018 to search for element 119. Curium was chosen as a target, rather than heavier berkelium or californium, as these heavier targets are difficult to prepare.[23] The 248Cm targets were provided by Oak Ridge National Laboratory, which had provided the necessary 249Bk target from the synthesis of tennessine (element 117) at Dubna. The RIKEN experiment began by being conducted at a cyclotron while it upgrades its linear accelerators, which resume operation in 2020. Bombardment may be continued with both machines until the first event is observed; the experiment is currently running intermittently for at least 100 days per year.[23][24] Hideto En'yo, director of the RIKEN Nishina Centre, predicted that elements 119 and 120 would probably be discovered by 2022.[25] The RIKEN team's efforts are being bankrolled by the Emperor of Japan.[26]
248 96Cm + 51 23V → 299 119Uue * → 296 119Uue + 3 1 0 n
248 96Cm + 51 23V → 299 119Uue * → 295 119Uue + 4 1 0 n
The produced isotopes of ununennium are expected to undergo two alpha decays to known isotopes of moscovium (288Mc and 287Mc respectively), which would anchor them to a known sequence of five further alpha decays and corroborate their production. The predicted cross section for these reactions is about 10 fb.[23]
Planned
Following the claimed synthesis of 293Og in 1999 at the Lawrence Berkeley National Laboratory from 208Pb and 86Kr, the analogous reactions 209Bi + 86Kr and 208Pb + 87Rb were proposed for the synthesis of element 119 and its then-unknown alpha decay daughters, elements 117, 115, and 113.[27] The retraction of these results in 2001[28] and more recent calculations on the cross sections for "cold" fusion reactions cast doubt on this possibility; for example, a maximum yield of 2 fb is predicted for the production of 294Uue in the former reaction.[29] Radioactive ion beams may provide an alternative method utilizing a lead or bismuth target, and may enable the production of more neutron-rich isotopes should they become available at required intensities.[29]
The team at the Joint Institute for Nuclear Research in Dubna, Russia, is planning to begin new experiments on the synthesis of ununennium using the 249Bk + 50Ti reaction in 2019 using a new experimental complex.[30][31][32][33][34][35]
The laboratories at RIKEN in Japan and at the JINR in Russia are best suited to these experiments as they are the only ones in the world where long beam times are accessible for reactions with such low predicted cross sections.[36]
How it was named?
Using Mendeleev's nomenclature for unnamed and undiscovered elements, ununennium should be known as eka-francium. Using the 1979 IUPAC recommendations, the element should be temporarily called ununennium (symbol Uue) until it is discovered, the discovery is confirmed, and a permanent name chosen.[37] Although widely used in the chemical community on all levels, from chemistry classrooms to advanced textbooks, the recommendations are mostly ignored among scientists who work theoretically or experimentally on superheavy elements, who call it "element 119", with the symbol E119, (119) or 119.
Predicted properties
Nuclear stability and isotopes
A chart of nuclide stability as used by the Dubna team in 2010. Characterized isotopes are shown with borders. Beyond element 118 (oganesson, the last known element), the line of known nuclides is expected to rapidly enter a region of instability, with no half-lives over one microsecond after element 121. The elliptical region encloses the predicted location of the island of stability.[11]
Orbital’s with high azimuthal quantum number are raised in energy, eliminating what would otherwise be a gap in orbital energy corresponding to a closed proton shell at element 114, as shown in the left diagram which does not take this effect into account. This raises the next proton shell to the region around element 120, as shown in the right diagram, potentially increasing the half-lives of element 119 and 120 isotopes.[38]
The stability of nuclei decreases greatly with the increase in atomic number after curium, element 96, whose half-life is four orders of magnitude longer than that of any currently known higher-numbered element. All isotopes with an atomic number above 101 undergo radioactive decay with half-lives of less than 30 hours. No elements with atomic numbers above 82 (after lead) have stable isotopes. Nevertheless, for reasons not yet well understood, there is a slight increase of nuclear stability around atomic numbers 110–114, which leads to the appearance of what is known in nuclear physics as the "island of stability". This concept, proposed by University of California professor Glenn Seaborg, explains why superheavy elements last longer than predicted.[40]
The alpha-decay half-lives predicted for 291–307Uue are on the order of microseconds. The longest alpha-decay half-life predicted is ~485 microseconds for the isotope 294Uue.[41][42][43] When factoring in all decay modes, the predicted half-lives drop further to only tens of microseconds.[1][7] Some heavier isotopes may be more stable; Fricke and Waber predicted 315Uue to be the most stable ununennium isotope in 1971.[2] This has consequences for the synthesis of ununennium, as isotopes with half-lives below one microsecond would decay before reaching the detector, and the heavier isotopes cannot be synthesised by the collision of any known usable target and projectile nuclei.[1][7] Nevertheless, new theoretical models show that the expected gap in energy between the proton orbitals 2f7/2 (filled at element 114) and 2f5/2 (filled at element 120) is smaller than expected, so that element 114 no longer appears to be a stable spherical closed nuclear shell, and this energy gap may increase the stability of elements 119 and 120. The next doubly magic nucleus is now expected to be around the spherical 306Ubb (element 122), but the expected low half-life and low production cross section of this nuclide makes its synthesis challenging.[38]
Being the first period 8 element, ununennium is predicted to be an alkali metal, taking its place in the periodic table below lithium, sodium, potassium, rubidium, caesium, and francium. Each of these elements has one valence electron in the outermost s-orbital (valence electron configuration ns1), which is easily lost in chemical reactions to form the +1 oxidation state: thus the alkali metals are very reactive elements. Ununennium is predicted to continue the trend and have a valence electron configuration of 8s1. It is therefore expected to behave much like its lighter congeners; however, it is also predicted to differ from the lighter alkali metals in some properties.[1]
The main reason for the predicted differences between ununennium and the other alkali metals is the spin–orbit (SO) interaction—the mutual interaction between the electrons' motion and spin. The SO interaction is especially strong for the superheavy elements because their electrons move faster—at velocities comparable to the speed of light—than those in lighter atoms.[44] In ununennium atoms, it lowers the 7p and 8s electron energy levels, stabilizing the corresponding electrons, but two of the 7p electron energy levels are more stabilized than the other four.[45] The effect is called subshell splitting, as it splits the 7p subshell into more-stabilized and the less-stabilized parts. Computational chemists understand the split as a change of the second (azimuthal) quantum number l from 1 to 1/2 and 3/2 for the more-stabilized and less-stabilized parts of the 7p subshell, respectively.[44][c] Thus, the outer 8s electron of ununennium is stabilized and becomes harder to remove than expected, while the
7p3/2 electrons are correspondingly destabilized, perhaps allowing them to participate in chemical reactions. This stabilization of the outermost s-orbital (already significant in francium) is the key factor affecting ununennium's chemistry, and causes all the trends for atomic and molecular properties of alkali metals to reverse direction after caesium.
Ununennium, 119Uue
Ununennium
Pronunciation
Alternative names
element 119, eka-francium
[315] (predicted)
Ununennium in the periodic table
Fr ↑ Uue ↓ (Ust) oganesson ← ununennium → unbinilium
Atomic number (Z)
119
group 1: H and alkali metals
Unknown chemical properties, but predicted to be an alkali metal
Electrons per shell
2, 8, 18, 32, 32, 18, 8, 1 (predicted)
Physical properties
903 K (630 °C, 1166 °F) (predicted)[2]
Density (near r.t.)
3 g/cm3 (predicted)[1]
Atomic properties
(+1), (+3) (predicted)[1]
Pauling scale: 0.86 (predicted)[4]
· 1st: 463.1 kJ/mol
· 2nd: 1698.1 kJ/mol
· (predicted)[5]
263–281 pm (extrapolated)[3]
Other properties
body-centered cubic (bcc)
(extrapolated)[6]
54846-86-5
History
Naming
IUPAC systematic element name
-Taken from Wikipedia
GANPAT 💩